Quantum Communications and Chinese SSBN Strategy
China’s quantum lead may have important implications for its SSBN force.
By Raymond Wang
November 04, 2017
China has been making significant advances in quantum technology, and it is important to understand the strategic implications of these developments as they enter military use. One area that merits close analysis is how quantum communications technology may influence the sea-based leg of China’s nuclear deterrent, the People’s Liberation Army Navy’s (PLAN) ballistic missile nuclear submarine (SSBN) force. Quantum technology has important implications for SSBN launch pre-delegation and patrol strategy. These implications are not alien to Chinese strategists and shipbuilders, who have already started interacting with top quantum physicists in China.
In short, quantum communication uses
photons to transmit information. As such, its underwater performance is affected by water quality. By comparing the relative clarity of the bodies of water surrounding China, certain areas appear to be significantly clearer than others, meaning that signals can penetrate deeper. This will influence ballistic missile submarine (SSBN) patrol strategy if quantum communication technology is deployed with the SSBN force. Furthermore, because of the nature of quantum mechanics, these communications are “
unhackable,” which will decrease technical pressures for launch authority pre-delegation, and increase crisis stability.
Quantum Communications and Water Quality
Enjoying this article? Click here to subscribe for full access. Just $5 a month.
Quantum communications use the quantum states of photons to transmit information. Photons are bundles of electromagnetic energy that make up light. The only principle required for this discussion is that photons are light of a particular wavelength, which affects their penetrative ability in different types of water.
The PLAN has long sought to improve its ability to securely communicate with SSBNs on patrols. It was
reported in 2013 that the PLAN’s Extremely Low Frequency (ELF, 30-300 Hz) communication systems went online, which represents the adoption of a “classical” submarine communications network using radio waves. ELF systems can communicate to around 100 meters in depth. Note that China defines 30-300Hz as Super Low Frequency (极低频/SLF), whereas the Institute of Electrical and Electronics Engineers (IEEE) defines it as ELF.
The next step toward more secure communications could be quantum communications. On August 30, 2017, the CEO of China Shipbuilding Industry Corporation, which produces China’s SSBNs,
met with Pan Jianwei.
Pan Jianwei is one of the leading quantum physicists in China. During the meeting, they reaffirmed cooperation on “quantum communication, quantum navigation and quantum sensing (radar).”
Recently,
a team of Chinese scientists successfully maintained the entangled state of a pair of photons through seawater by developing a method of “filtering out” depolarized photons at the receiving end. This represents a significant step toward developing a quantum communication capability for Chinese SSBNs.
The scientists from the study chose water samples from six points spanning 36 km in the Yellow Sea, which
happens to be one of the areas identified by Chinese analysts as a probable site for Chinese SSBN patrols. There are also technical reasons for choosing the Yellow Sea. The
authors remark that the water there was Jerlov type I, which is the clearest category under the Jerlov water classification scheme.
This shows that the adoption of quantum technology would introduce water quality as a new factor that will affect the capabilities of a submarine communications network. To be sure, any quantum underwater communication will experience attenuation — signal loss due to travel in water — and it is reasonable to assume there will be measures to compensate for such problems. However, it is also reasonable to assume that even with such measures, there will still be an attenuation threshold at which quantum communications become unfeasible. Certain quantum communication protocols can
tolerate up to 70db of loss, but there is currently no available open-source information to determine the kind of protocol that will be used in such an SSBN communication system.
The Jerlov scheme classifies seawater into different types, each with its distinct attenuation coefficient (Kd) curve. Kd is inversely proportional to the Z90 value (Kd = 1/Z90), which represents the depth at which 90 percent of a signal is lost.
In a nutshell, the lower the Kd value, the clearer’the water, and the deeper a quantum signal can penetrate. Another property of water types is that its clarity for light at different wavelengths is transferable – that is, if water type A is clearer than water type B at a given wavelength, then it is clearer than type B at
all wavelengths.
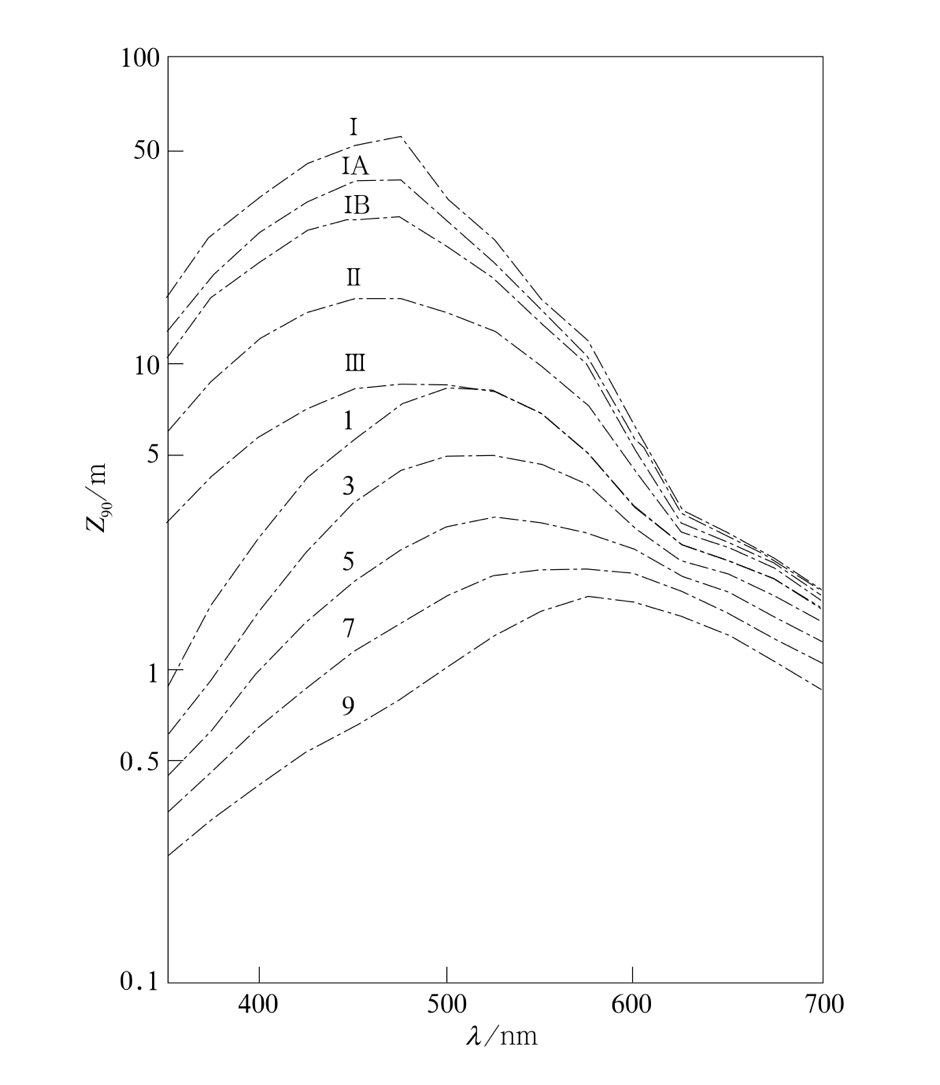
Figure 1 Variation of Z90 with wavelength, taken from Liu Ying & Li Guosheng, Study of attenuation depths for MODIS bands in the Bohai Sea in China, Acta Oceanologica Sinica 2009, Vol. 28, No. 5, p. 44.
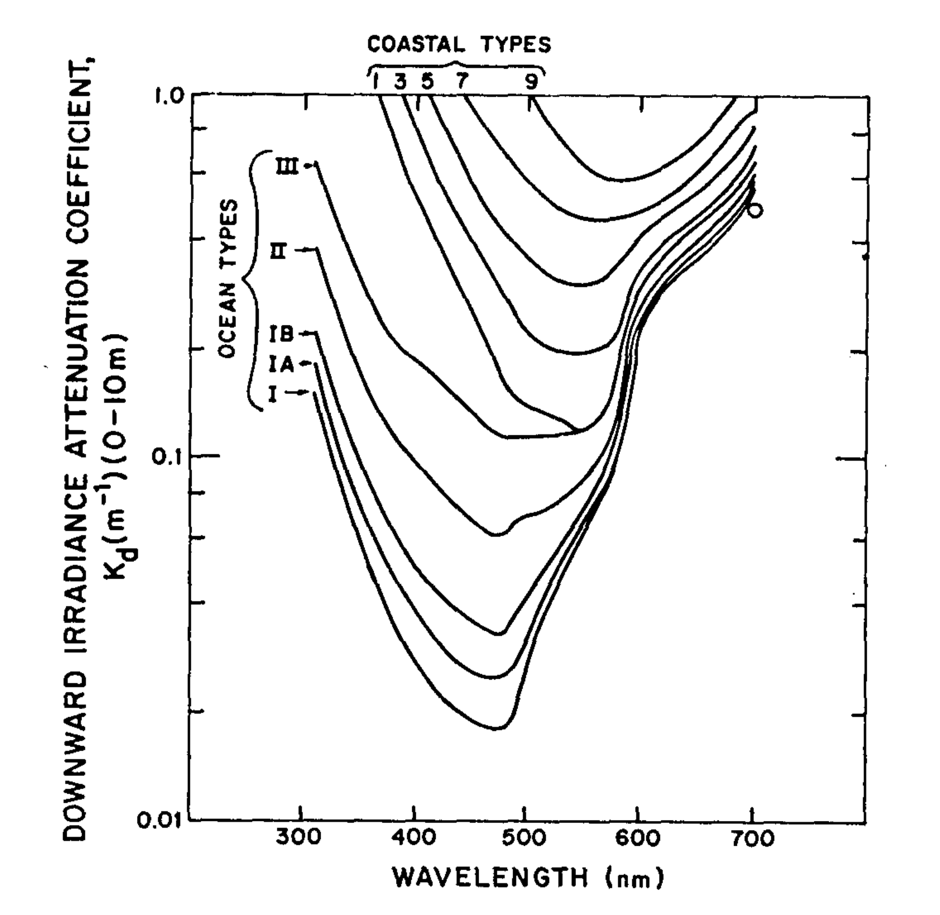
Figure 2 Variation of Kd with wavelength, taken from Northam, Donna et al, High repetition rate frequency-doubled Nd: YAG laser for airborne bathymetry, Applied Optics, Vol. 20, No. 6, 1981, p. 969.
SSBNs can patrol in “murky” areas and go to “clear” areas to receive signals. Alternatively, they can use buoyant antennas similar to the ones used to receive VLF signals — this would allow the SSBN to remain submerged at its operating depth, while trailing an antenna that floats to a depth with stronger signal. However, the Chinese ELF
report observes that this will “limit the SSBN’s speed to 4 knots, and there is still a chance that the antenna could be detected.” This suggests that, doctrinally, the PLAN hopes to move away from using buoyant antennas. However, very clear water with around 0.01Xm-1 Kd is very rare, especially in coastal regions. Indeed, even at the clearest points identified in the analysis below, the average clarity would only translate to a Z90 of around 40 meters, which means only 10 percent of the quantum signal would remain at 40 m. As such, despite its doctrinal reluctance, the PLAN may have to occasionally deploy buoyant antennae. This will face the same issues of water quality, unless the antenna goes above the surface; something the PLAN would hope to avoid.
It is beyond the scope of this article to take into account the effect of the air-water interface, an important factor for which I am indebted to Dr. Richard Garwin. Suffice it to say that makes sending signals accurately difficult due to the refractive properties of seawater, especially when taking surface ripples into account. One interesting consequence is that it will be much harder for the SSBN to send signals accurately back to the satellite than
vice versa, as the signal has to travel farther to reach the satellite than the SSBN, therefore magnifying any deviations resulting from the refractive effect.
Analysis of the Four Bodies of Water
Figure 3 is taken from a study that used remote sensing data to calculate the Kd values at a signal wavelength of 490 nm. It is common practice to denote the Kd value at a particular wavelength as Kd(wavelength). The study encompasses the Bohai Gulf, the Yellow Sea, and the East China Sea. These results were compared with
otherstudies that collected
in situ data from the Bohai Gulf and the Yellow Sea, and proved to be consistent. Note that the Chinese scientists in the quantum study used photons at a wavelength of 405 nm, and Kd values in the 400-500 nm range increase as the wavelength decreases. As such, Kd(405) values would be slightly higher than the ones discussed below, which are measured at Kd(490).
Bohai Gulf and Yellow Sea
Figure 3 shows that the Bohai Gulf is not a favorable area for quantum communications, with Kd(490) values mostly at around 1.00 m-1, meaning 90 percent of the signal will be lost at 1 m. The Yellow Sea is clearer, with lower Kd(490) values between 0.01 – 0.1 m-1. China would probably prefer to conduct patrols in the northern part of the Yellow Sea, defined as the area above the 38th parallel, despite it being slightly murkier than the area below. This is because the northern part is bounded by the Chinese and North Korean border, whereas the latter would put Chinese SSBNs close to areas that likely contain South Korean and U.S. anti-submarine assets.
East China Sea
The East China Sea is significantly affected by the discharge of the Yangtze River, which carries a lot of sediment. The coastal areas surrounding the outlet have extremely high Kd values going up to 6.00m-1, meaning 90 percent signal loss at 0.17 m. The “plume” effect of this discharge affects a large part of the coastal area of the East China Sea, and in order to get to comparable water qualities (light blue/deep blue), SSBNs would have to go to areas close to the South Korean Jeju Island, or away from the coast in the southeastern part, where Japan and the United States “
most likely deploy a variety of anti-submarine assets.”

Figure 3 Adapted from Son and Wang using the annotation tool szoter.com, The Diffuse Attenuation Coefficient Model in the Yellow Sea for the Korean Geostationary Ocean Colour Imager, Proceedings of SPIR, Vol. 7861, p. 5.
South China Sea
The northern South China Sea encompasses Hainan Island, where the South Sea Fleet is based
at Yulin Naval Base. Figures 4 and 5 are taken from a
study comparing the coastal waters of Guangdong and the northern South China Sea (NSCS), and demonstrate that the waters south of Hainan Island have low Kd values while being close to the Chinese coast, and is also the
deepest among the four bodies of waters. The Kd values in northern South China Sea remain close to 0.01 from l=400-500 nm, as shown in Figure 5.
More specifically, it determines that Kd(490) at the NSCS range from 0.034-0.193 m-1, with an average of 0.073 m-1, with most samples less than 0.092 m-1. This is reflected in the one outlier data line for the NSCS in Figure 5. Using an empirically derived formula provided in the study, Kd(412) can be estimated to be 0.018m-1 at the clearest point in the NSCS, representing 90 percent signal loss at around 55 m. We can expect Kd(405) to be around this number. This gives a sense of the relative clarity of the NSCS.
As such, should the PLAN adopt quantum technology and a coastal patrol strategy, the northern South China Sea and the northern Yellow Sea are the most favorable areas. The PLAN will likely adopt a coastal patrol strategy for the near future, given the noisy nature of its Type 094 SSBN. At the time of writing, the PLAN has four active Type 094 SSBNs in service, all
based at the Yulin base in Hainan.
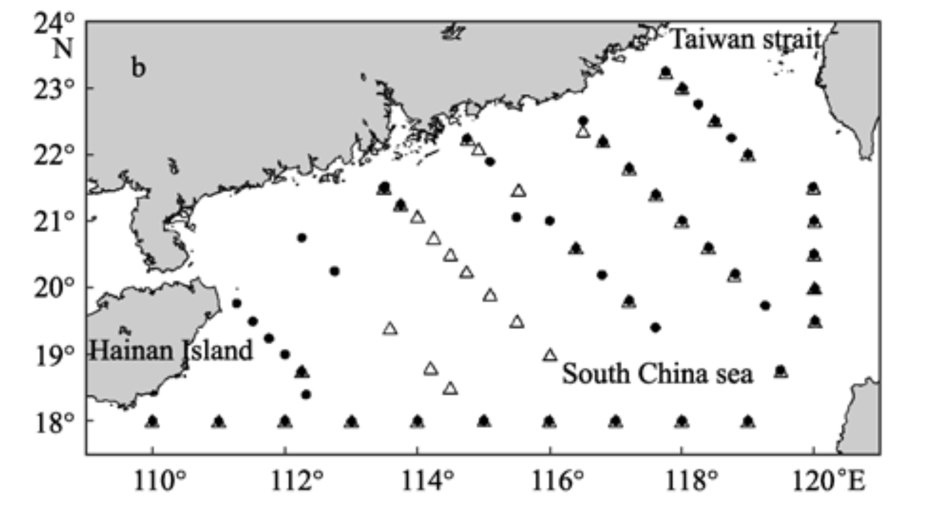
Figure 4 Sampling stations in the northern South China Sea in 2004(•) and 2005(Δ), taken from Wang et al,. Variation in downwelling diffuse attenuation coefficient in the northern South China Sea p. 3.
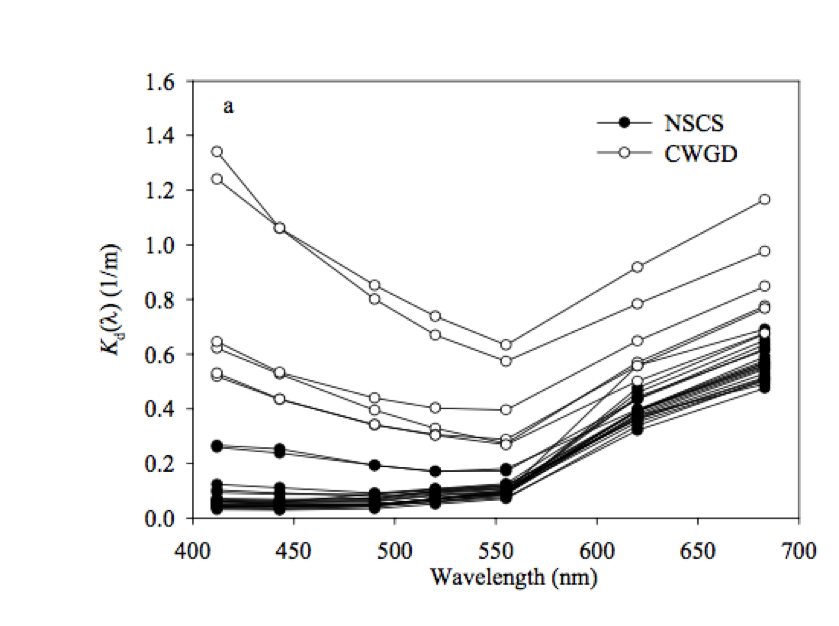
Figure 5 Kd values of NSCS cruises, taken from Wang et al., Variation in downwelling diffuse attenuation coefficient in the northern South China Sea p. 4.
This analysis leads to one more observation: since oceanic waters generally have lower Kd values than coastal waters, adopting quantum communications channels implies a trade-off – in order to get to clearer waters, submarines have to move farther away from the coast, i.e., farther away from the protection coverage of friendly land-based assets.
Quantum Key Distribution (QKD) or Pure Quantum Channel
There are two methods of deploying quantum communication channels. QKD is a hybrid model in which the message is transmitted via a classical channel but is encrypted with a key, which is then transmitted via a quantum channel. There are various QKD protocols, but the photon signal will be affected by water quality regardless of which is used.
A QKD model for land-to-submarine communications could be: the message would be transmitted via the Extremely Low Frequency (ELF) classical channel, and the key would be transmitted via a quantum channel, such as through a satellite. Attaining the shared key will require a “public” discussion on the classical channel. China
has already successfully used the Micius satellite to entangle photons and transmit each twin to two receiving stations on land. More recently, it was
used to make the first intercontinental quantum phone call from China to Austria, proving the feasibility of the world’s first space-to-ground integrated quantum network.
Under this model, China would still face
some challenges associated with using ELF, such as low bandwidth and the need to use large antennas, which would make such sites vulnerable targets. However, due to quantum encryption, the channel would be secure in the sense that the message, that is the key, in the quantum channel cannot be read, making SIGINT collection by adversaries impossible. Due to the very nature of quantum mechanics, any attempt at eavesdropping on the channel would introduce “
disturbances into the system… and cause the quantum state of the key to change, and the information being intercepted will self-destruct.” This clarifies a popular misconception as to the reason quantum channels are “unhackable.” It is impossible to read an intercepted message, as the very act of intercepting will change the state of the message. However, it is still possible to
interrupt a quantum channel and thereby block a message, although the interruption will be detected.
A pure quantum channel solves problems related to a classical ELF channel, and has the same level of quantum security. It is possible that China can eventually produce such a channel, given a
recent experimental successusing direct counterfactual communication, which transmits a quantum state without any particles being physically sent between the two parties. However, the set-up from the experiment cannot utilize “a strong coherent state (classical) light,” and there is not enough available information to assess how this could be operationalized. In contrast, the aforementioned space-to-ground model is an existing operationalized QKD system. Therefore, a QKD system is a likely next step.
Both models will offer China increased confidence in the security of their SSBN communications. This would decrease technical pressure to pre-delegate launch authority, and increase crisis stability.
Crisis stability in part depends on both sides believing that there are no significant advantages to be gained by initiating nuclear war. Without QKD, the Chinese leadership might worry that at some point in a crisis, its commands can be spoofed or altered to cancel or interfere with SSBN launches, creating a “use it or lose it” scenario where there may be incentives to launch early. With QKD, they can be assured that messages will not be spoofed, altered, or read, thereby removing this pressure. This security will also reduce pressures to pre-delegate launch authority. That said, since QKD still requires ELF facilities, Chinese perceptions of their vulnerability to counterforce strikes — strikes by an adversary to destroy ELF facilities — will still affect crisis stability.
Policy Implications and Conclusions
This analysis shows that water quality will become a new factor in underwater communications. A technical and strategic analysis of the waters surrounding China show that the northern Yellow Sea and the northern South China Sea are more favourable toward quantum communication. This leads to a few policy implications.
First, water quality is by no means the only factor influencing the area of deterrence patrols. Other factors such as depth and salinity, which affect the detectability of SSBNs, will also play a role. SSBNs could patrol in murky areas and regularly go to clear areas to receive signals. That said, knowing certain areas are overall more favorable toward quantum communications would create “watering holes,”, whereby monitoring clear areas will give a good probability of locating an SSBN. It is also important to remember that water quality is affected by seasonal changes and other variables.
Second, the fact that oceanic waters generally have lower Kd values increase incentives to achieve open water patrols. This partially depends on when China will develop a quieter SSBN.
Third, as mentioned in the previous section, the adoption of quantum communications will increase confidence in the security of submarine communications, which will decrease technical pressures to pre-delegate launch authority, and increase crisis stability.
In the final analysis, developments in underwater quantum communications are a significant technological breakthrough. Further research in this area is needed to determine its full strategic implications.